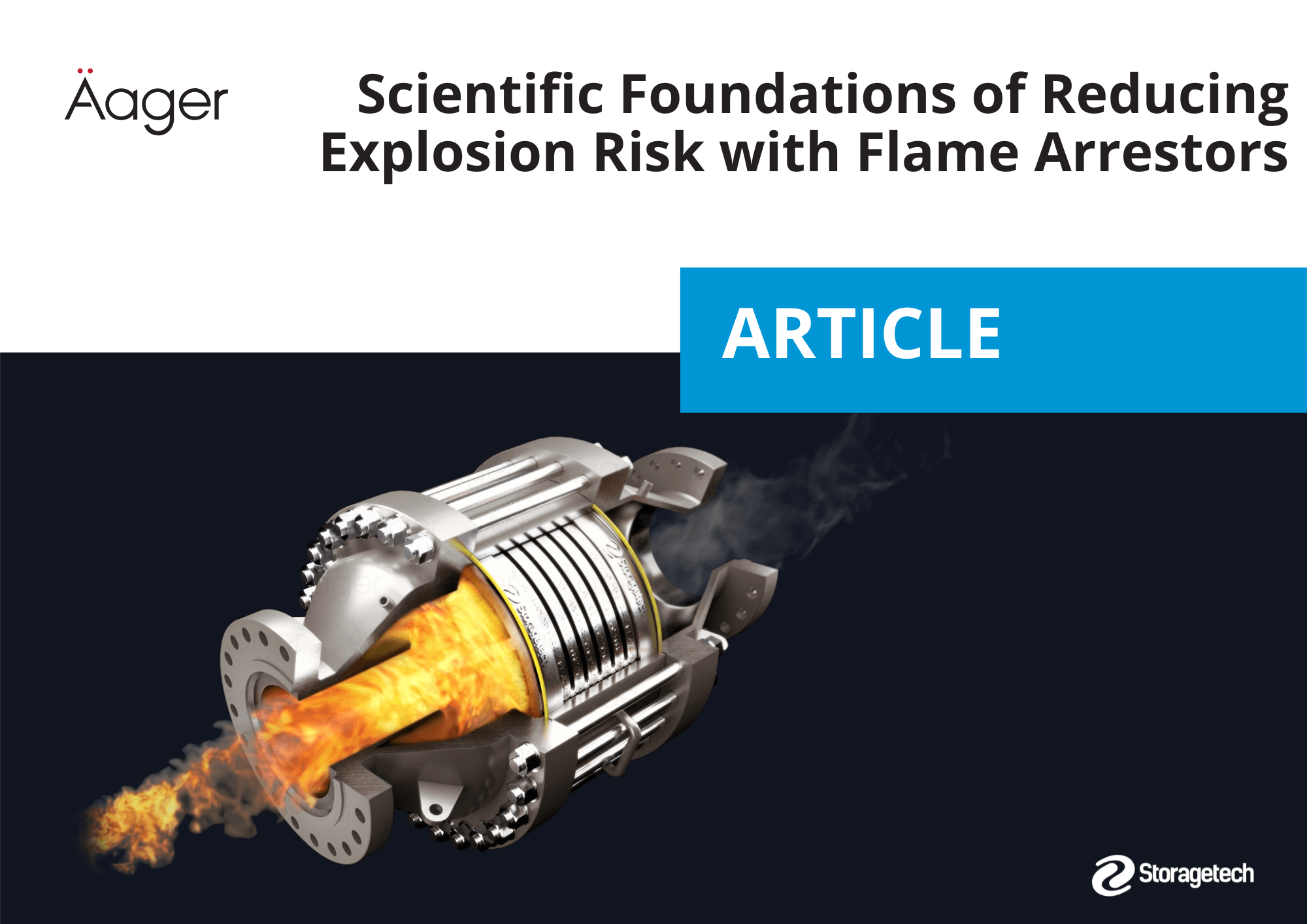
In the oil, gas, and chemical industries, the use of flammable and explosive materials makes advanced safety measures an absolute necessity. One of the most critical components of these safety measures is the flame arrestor, a device that prevents flames from traveling from one section of a system to another in potentially explosive environments. But what is the scientific principle behind these devices, and how do they reduce explosion risk?
1. Dynamics of Explosions and Flame Propagation
Fires or explosions occur when a flammable substance comes into contact with oxygen and an ignition source. Three main factors heighten the risk of an explosion:
1. Proper Fuel-Air Mixture
- When flammable gases or vapors are present in certain concentration ranges (LEL–UEL) with air, they become explosive.
2. Ignition Source
- Electrical sparks, static electricity, hot surfaces, and mechanical friction can all supply enough energy to ignite a flame.
3. Pressure and Velocity
- In pressurized environments, the flame front can accelerate, increasing the potential destructiveness of an explosion.
When these factors converge, the “flame front” spreads rapidly. Its progression speed can be categorized as either deflagration (subsonic) or detonation (supersonic).
2. Core Operating Principle of Flame Arrestors
Flame arrestors reduce explosion risk by eliminating the heat transfer needed for a flame to continue propagating. Their scientific basis lies in the principle of thermal quenching:
1. Heat Transfer
- The internal mesh or narrow pathways inside the flame arrestor restrict the flame’s path.
- Metal components, known for high thermal conductivity, absorb the heat energy carried by the flame.
2. Temperature Reduction
- For a flame to keep burning, the surrounding temperature must stay above the fuel’s ignition point.
- As the flame moves through the flame arrestor, it rapidly loses heat, dropping below the ignition temperature and thus cannot continue.
3. Pressure Stabilization
- When flame speed increases, pressure surges can intensify. The flame arrestor’s narrow channels help moderate these pressure fluctuations and flow conditions.
Through these mechanisms, the device effectively blocks the flame and prevents it from spreading to tanks, piping, or other equipment—reducing the risk of large-scale accidents. Overall, the thermal conductivity, geometry, and design of the flame arrestor determine its flame-quenching capacity.
3. Scientific Basis of Thermal Quenching
The ability of flame arrestors to absorb heat and extinguish a flame is rooted in the fundamental principles of thermodynamics and heat transfer:
- Fourier’s Law of Heat Conduction
The amount of heat conducted is dependent on the material’s thermal conductivity, the temperature gradient, and the cross-sectional area through which heat flows. - Convection and Radiation Effects
Part of the flame’s energy is dispersed through convection and radiation. Flame arrestors are designed to limit both forms of heat transfer effectively. - Temperature Threshold
Combustion stops when the temperature falls below the ignition point of the fuel. By interrupting the flame’s energy flow, the flame arrestor ensures that critical heat thresholds are not met.
4. Protection Against Deflagration and Detonation
Whether a flame front progresses as a deflagration (subsonic) or detonation (supersonic) significantly impacts the severity of an explosion. Flame arrestors are generally categorized by the type of flame they are designed to handle:
- Deflagration Flame Arrestors
- Intended for lower flame speeds and pressures (subsonic).
- Mesh or honeycomb structures effectively extinguish flame fronts under these conditions.
- Detonation Flame Arrestors
- Suitable for higher pressures and supersonic flame speeds (detonation).
- Possess layered and more robust designs capable of withstanding intense pressure shocks.
Though both types operate on the thermal quenching principle, they differ in mechanical resilience and the extent of surface area required for heat transfer.
5. Scientific Importance of Material Selection
The material used to manufacture flame arrestors directly influences how efficiently the device can absorb and dissipate heat, as well as resist corrosion. In chemically active environments, material choice is especially critical:
- High Thermal Conductivity Metals
Rapidly absorb the heat energy from the flame, bringing temperatures below ignition points. - Corrosion Resistance
In acidic, alkaline, or sulfur-rich environments, stainless steel or specially coated alloys prevent corrosion. - Mechanical Strength
For detonation scenarios, the flame arrestor body must withstand high pressures without deforming.
Proper material selection and design are guided by thermodynamics and fluid mechanics. Poor material choice or insufficient thickness can increase explosion risk or lead to excessive overheating.
6. Achieving Effective Results in Practice
Several practices can enhance the scientific effectiveness of flame arrestors:
- Accurate Design and Sizing
- Select models according to flow rate, pressure, temperature, and the possibility of deflagration or detonation.
- Ensure the device can handle worst-case scenarios.
- Routine Maintenance and Cleaning
- Accumulated deposits can block heat transfer surfaces.
- Regular cleaning ensures that the flame-quenching capacity remains at its highest.
- Certification and Standards
- Adherence to international standards (e.g., API, NFPA) indicates that the device has been rigorously tested under real-world conditions.
- Review technical data and test reports from the manufacturer.
- System Integration
- Combine flame arrestors with pressure safety valves, rupture disks, and inert gas systems for multi-layer protection.
- Such integration addresses unexpected pressure spikes and chemical reactions.
Highlights
- Thermal Quenching Mechanism: The foundational scientific principle of flame arrestors is the absorption of heat to extinguish a moving flame.
- Thermodynamics at Work: Fourier’s Law, as well as convection and radiation, are central to the device’s functionality.
- Material and Design: The thermal conductivity and mechanical integrity of metals are key to explosion prevention.
- Deflagration vs. Detonation: Different flame speeds require variations in flame arrestor design and construction.
When flame arrestors are properly selected, installed, and maintained, they serve as a crucial safeguard against large-scale fires and explosions. Grounded in robust scientific principles, they protect both human life and infrastructure by halting flames at the source and preventing them from traveling to critical process areas.
Mohammed HASSAN
Technical Coordinator
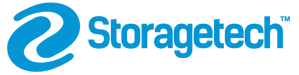